9. Types of Separation Modes
When selecting an HPLC column, if your target sample contains only few analytes with almost no impurities, only requirement for a column would be to elute all analytes from the column. This leaves a wide range of column selection. However, if your target sample contains multiple analytes and impurities, it is necessary to select a column that not only allows the analytes to elute from the column, but also allows the analytes to be separated from each other and from the impurities. Therefore, the first step in column selection is selection of separation mode. In “5. Principle of Separation”, we explained “separation is possible if there is a difference in degree of interaction”. This can be rephrased as “separation is possible by taking an advantage of differences in analyte and impurity’s properties”. Table 1 summarizes the property differences used in each separation mode.
Table 1 Property differences used in each separation mode
Separation Mode | Property Differences |
---|---|
Reversed, Normal, Hydrophilic Interaction Chromatography (HILIC) | Polarity |
Ion exchange, ion exclusion | Ionic characteristics |
Ligand exchange | Ligand exchange ability |
Size exclusion chromatography (SEC) | Molecular size (molecular weight) |
Optical splitting, Affinity | Specificity |
Multimode | Combination |
From here, we will explain separation mechanisms of each separation mode.
10. Separation by Polarity
We will start with explaining separation modes that use component’s polarity differences. Expressions such as high polarity and low polarity are used to indicate the polarity of a substance. Expressions such as hydrophilicity and hydrophobicity are also often used to mean the same. Hydrophilicity indicates a substance’s ease of water solubility, and hydrophobicity indicates a substance’s difficulty in water solubility. We can also say that "high polarity" is equal to "highly hydrophilic" and "low polarity" is equal to "highly hydrophobic".
10-1. Reversed phase mode (reversed phase chromatography) and its mechanism
Reversed phase mode is a separation mode that takes an advantage of polarity differences in-between analytes. It is said that over 80% of HPLC use reversed-phase mode, making it the most commonly used separation mode. In reversed-phase mode, a packing material with a low surface polarity and an eluent of a highly polar solvent (mixtures of water/acetonitrile, water/methanol, etc.) are used. The analyte’s partition between the packing material and eluent is influenced by their polarities, where the influence of packing material polarity is stronger than eluent polarity. For this reason, the more polar the analyte, the greater the distribution in eluent, that is the faster the movement speed within the column, and the faster it elutes from the column. On the other hand, elution of analyte with low polarity takes a longer time because of its large distribution in the packing material. Therefore, in reversed phase mode, analytes are eluted in an order of higher polarity to lower polarity. Figure 14 shows reversed phase mode separation mechanism, and figure 15 shows an elution order of alkyl alcohols in reversed phase mode. It shows that the larger the alkyl chain (red) of the alcohol, the slower the elution.
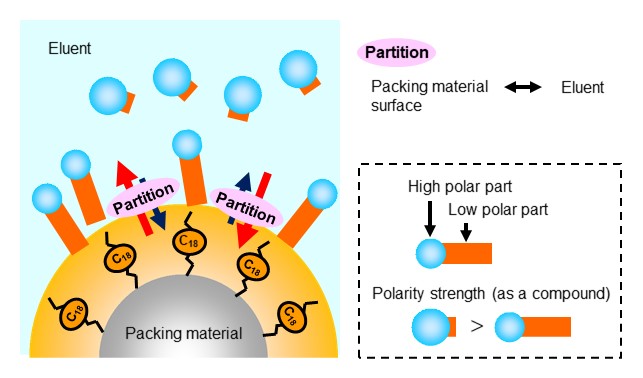
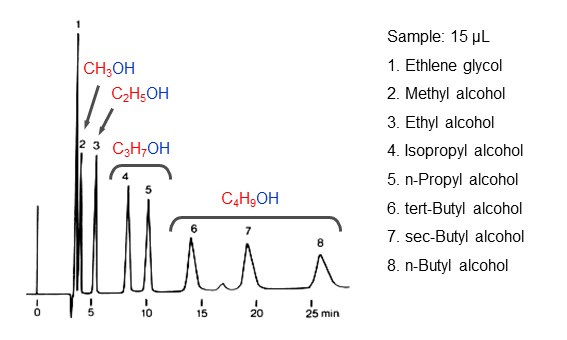
- Column
- : Shodex RSpak DE-613 (6.0 mm I.D. x 150 mm)
- Eluent
- :H2O
- Flow rate
- :1.0 mL/min
- Detector
- :RI
- Column tamp.
- :60 ℃
ODS column
ODS columns are the major reversed phase mode columns. As shown below, an ODS column is filled with silica-base packing material modified (bonded to the particle surface) with linear alkyl group having 18 carbon atoms (octadecyl group). ODS columns are named after the bonded compound, “octa-decyl-silyl”. The bonding reaction takes by letting octadecylsilane reacts with silanol groups on the surface of silica base material, and this reaction is called sialylation. Among silica-based packing materials, ODS columns cover an impressively large proportion, and it is no exaggeration to say that they are the most used HPLC columns.

End-capping
When attaching functional groups to the silica base material, not all silanol groups can be attached and some silanol groups remain unsilylated. The silanol groups that remain without reacting are called residual silanol groups. Residual silanol groups can cause unexpected secondary interactions with some analytes and may affect separation. Normally, the remaining silanol groups are subjected to a secondary silylation process called end-capping to prevent secondary interactions from occurring. However, it is known that even the end-capping is difficult to completely silylate all remaining silanol groups. Residual silanol groups are one of the reasons why analytical results may differ among the column used, even they are all ODS columns. Although we will omit the explanation here, ODS columns have monomeric and polymeric bonding types, and the separation characteristics change depending on this difference also.
Other silica base reversed phase columns
As mentioned, ODS columns are the most common reversed phase mode columns. In some cases, retention of target analytes is too strong with octadecyl (C18) groups. This retention can be weakened by changing the linear alkyl functional group choice to shorter carbon chain ones. An example is shown below.
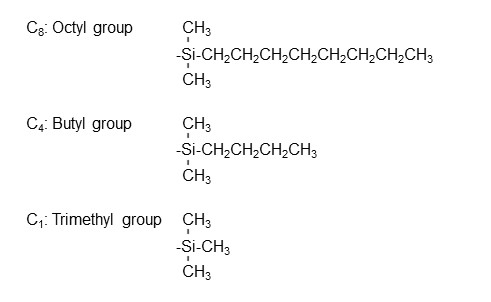
Other available reversed phase columns include the ones modified with phenyl and cyanopropyl groups.
Polymer base revered phase columns
There are reversed phase columns using polymer-based packing materials. Some characteristics of the polymer-based reversed phase columns are listed below.
- (1)Durability
Silica-based packing materials undergo hydrolysis during use or storage, often resulting in removal of their functional groups. On the other hand, polymer-based materials have high chemical bond stability and durability. - (2)Reproducibility
It is difficult to control gel-to-gel lot quality of silica-based packing material. Compared to the silica-based material, polymer-based materials are easier to control the manufacturing process and maintain stable product quality.
Please check Features of RSpak DE Series (4) (Reproducibility between Production Lots) - (3)Alkali durability
In general, silica-based materials have low alkali durability. Alkaline solvents are sometimes effectively used as an eluent for basic compound analysis. For those cases, polymer-based materials with high alkali durability are an advantage. Additionally, alkaline washing may be effective when the separation performance of a column deteriorates due to impurities being adsorbed to the packing material. Silica-based columns cannot be washed with alkali solvents and so the column must be replaced. Meanwhile, polymer-based columns can be washed with alkaline solvents, which may increase a possibility of regenerating the column.
Please check Repeatability for ODP-50 4E, A Polymer-based Column for Reversed Phase Chromatography, under Alkaline Conditions and Advantage of ODP2 HP (5) Alkali Durability - (4)Separation efficiency
Generally, polymer-based columns have slightly lower separation efficiency than that of silica-base columns. However, the separation efficiency of polymer-based columns had continuously been improving also.
Shodex has an extensive expertise in polymer synthesis and have been developing various polymer-based reversed phase columns. Among them, the most widely used is the Asahipak ODP series. We named "ODP" after octadecyl group (octadecyl) modified polymer base material (polyvinyl alcohol gel), hoping to make users easily recognize it as an alternative choice of familier ODS columns.
Shodex offers a range of polymer-based reversed phase columns that utilize low polarity of polymer base material itself. Many of our polymer-based reversed phase columns are not modified with functional groups. Each offer different surface polarity and pore sizes.
10-2. Normal phase mode (normal phase chromatography) and its mechanism
Next, we will explain normal phase mode. Similar to reversed phase mode, normal phase also takes an advantage of polarity differences in-between analytes. In reversed phase mode, a packing material with a high surface polarity (e.g., silica gel by itself) and an eluent of non-polar solvent (mixtures of hexane and isopropyl alcohol etc.) are used. The analyte’s distribution between the packing material and eluent is influenced by their polarities, where the influence of packing material polarity is stronger than the eluent polarity. Opposite to the reversed phase elution sequence, analytes with lower polarity will move faster. Therefore, in normal phase mode, analytes are eluted in an order of lower polarity to higher polarity.
10-3. HILIC mode (hydrophilic interaction liquid chromatography) and its mechanism
HILIC stands for Hydrophilic Interaction liquid Chromatography. An American scientist A. J. Alpert introduced the idea of HILIC in 1990. HILIC mode belongs to normal phase mode in a broad sense. Historically, the technique itself has been used as normal phase mode for sugar analysis and other applications even before 1990. In HILIC mode, a packing material with a highly polar surface is used, such as amide groups, amino groups, and diol functional groups are bonded to silica or polymer base materials. Like a reversed phase mode, an eluent used is a highly polar solvent such as a mixture of water and acetonitrile, with higher organic solvent ratio. Under this condition, a hydration layer forms on the packing material surface. The analyte’s distribution between the packing material and eluent is influenced by their polarities, where the influence of packing material polarity is stronger than the eluent polarity. In HILIC mode, analytes are eluted in an order of higher polarity to lower polarity. i.e., you may memorize that the eluent of HILIC mode is similar to the normal phase mode and the elution order is opposite to reversed phase mode. In HILIC mode, in addition to partitioning, electrostatic interactions, hydrogen bonding, and van der Waals interactions work in a complex manner. Figure 16 shows HILIC mode separation mechanism, and figure 17 shows elution order of maltooligosaccharides in HILIC mode. Maltooligosaccharides are oligosaccharides in which glucose is linked with α-1,4 glucoside bonds. Glucose has multiple hydroxyl groups (OH groups) in its structure, and the more glucose it binds, the more polar it becomes. It can be seen that the larger the sugar chain of the oligosaccharides, the slower the elution.
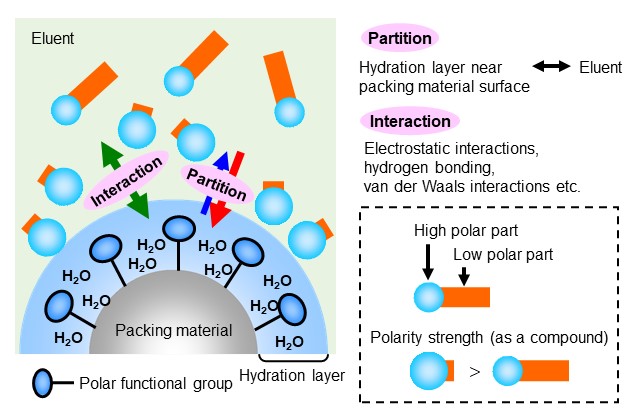
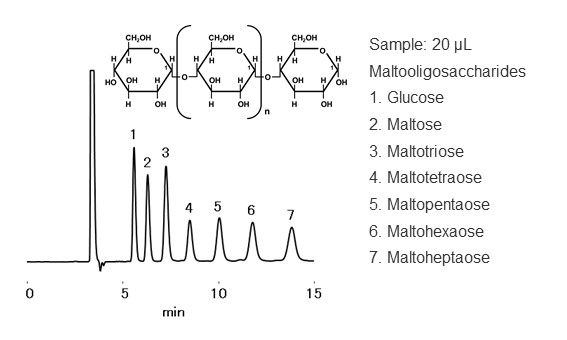
- Column
- :Shodex HILICpak VG-50 4E (4.6 mm I.D. x 250 mm)
- Eluent
- :CH3CN/H2O=65/35
- Flow rate
- :0.7 mL/min
- Detector
- :RI
- Column tamp.
- :40 ℃
Figure 17 Elution order of maltooligosaccharides in HILIC mode
HILIC mode allows analyses of highly polar compounds that are weakly or difficult to retain by reversed phase mode. Taking an advantage of this feature, HILIC mode is used for analyses of various high polar compounds, which include sugar analysis. Shodex is offering a polymer-based amino functional group HILIC column series, Asahipak NH2P, and polymer-based columns modified with various functional groups, HILICpak series HILIC columns. These HILIC mode columns, like polymer-based reversed phase mode columns, have high chemical bond stability and excellent alkali durability. Table 2 summarizes a comparison of reversed phase, normal phase, and HILIC modes.
Table 2 Comparison of reversed phase, normal phase, and HILIC modes
Separation Mode | Reversed Phase Mode | Normal Phase Mode | HILIC Mode |
---|---|---|---|
Packing Material Surface | Low polar | High polar | High polar |
Eluent | High polar | Low polar | High polar |
Elution Order of Analytes | High polar to low polar | Low polar to high polar | Low polar to high polar |
Target Analyte | Drugs, surfactants, peptides, water-soluble vitamins, etc. | Fatty acids, phospholipids, fat-soluble vitamins, etc. | Sugars, oligonucleic acids, amino acids, peptides, pharmaceutical metabolites, etc. |
Features |
・Main targets are low molecules with molecular weight 2,000 or less |
・Main targets are low molecules with molecular weight 2,000 or less |
・Main targets are low molecules with molecular weight 2,000 or less |
Find Shodex Reversed Phase and Hydrophilic Interaction Chromatography Columns HERE
11. Separation by Ionic Differences
Just as the North and the South poles of a magnet attract each other, positively charged cations and negatively charged anions attract each other. Similarly, as between the North and the South poles, cations and anions repel each other. Ionic substances exist in a wide variety and forms. They include biological substances such as proteins, peptides, amino acids, and catecholamines, organic substances such as organic acids, and inorganic ions such as chloride ions (Cl-) and sodium ions (Na+). In this section, we will introduce two related modes: ion exchange mode, which uses attraction forces between ions (electrostatic interaction) and ion exclusion mode, which uses repelling forces between ions (electrostatic repulsion).
11-1. Ion exchange mode (ion exchange chromatography) and its mechanism
Using anionic substance analysis as an example, we will explain the separation mechanism of ion exchange mode. The packing materials for anion analyses are modified with cationic functional groups. Buffers and salt solutions are typically used as an eluent in ion exchange mode. Figure 18 shows steps of ion exchange separation.
- (1) Anions in an eluent adsorb to cations of functional groups through electrostatic interactions. As the eluent passes through the column, anions in the eluent compete with each other and move through the column with continuous adsorption and desorption.
- (2 to 3) When sample analytes (anionic substances) enter into the column, they compete with anions from the eluent and adsorb to the functional group.
- (4 to 5) Analytes move through the column in competition with anions in the eluent (repeated adsorption and desorption).
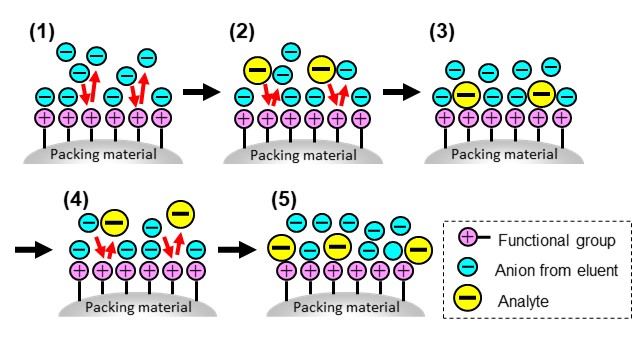
As analytes move through the column while exchanging the adsorption sites with competing ions in the eluent, the higher the ionic strength of the eluent, the weaker the retention of the analytes become. In ion exchange mode, it is common to use "gradient elution," which changes the eluent composition over time in order to elute highly retained analytes faster. A typical gradient elution is a condition in which the salt concentration is increased over time. In ion exchange mode, the higher the analyte’s charge or the smaller the analyte’s hydrated ionic radius, the stronger the affinity with the functional group. Thus, in general, the elution order of analytes is from ions with lower charges or larger hydrated ionic radii to ions with lower charges or smaller hydrated ionic radii. Table 3 summarizes the functional groups used in ion exchange chromatography.
Table 3 Functional groups used in ion exchange chromatography
Separation Mode | Anion Exchange Mode | Cation Exchange Mode | ||
---|---|---|---|---|
Functional Group Type (Ion Exchange Resin) |
Strong anion exchange group (Strong basic ion exchange resin) |
Weak anion exchange group (Weak basic ion exchange resin) |
Strong cation exchange group (Strongly acidic ion exchange resin) |
Weak cation exchange group (Weak acidic ion exchange resin) |
Common Functional Group | Quaternary ammonium group (QA) | Diethylaminoethyl group (DEAE) | Sulfopropyl group (SP) |
Carboxylmethyl group (CM) |
Target Analyte | Anionic substance | Cationic substance |
Eluent pH affects the dissociation state of analytes and functional groups. Strong ion exchange groups dissociate regardless of the eluent pH, so we only need to consider dissociation state of analytes. However, with weak ion exchange groups, the dissociation state changes depending on the eluent pH. The dissociation states of both functional group and analytes need to be taken into an account.
11-2. Protein analysis
Proteins are substances made up of various amino acids bounded together by amide bonds. As shown in figure 19, proteins are zwitterionic substances that have cations and anions in their structures. Particular care must be taken when analyzing such zwitterionic compounds. It is important to pay an attention on isoelectric points (pI) of target proteins when selecting a column, especially when analyzing multiple proteins together. Proteins are positively charged in solutions with a pH lower than their isoelectric point (pI) and negatively charged in solutions with a higher pH. The ion exchange mode column to be selected depend on the pH of the eluent to be used.
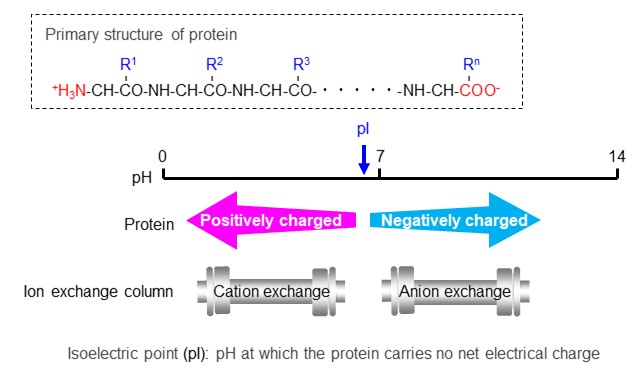
Figure 20 shows the elution order of standard proteins using strong anion mode (functional group: quaternary ammonium group). In pH 6.0 eluent, conalbumin has a positive charge and other proteins have a negative charge. The positive charge of conalbumin is the same as packing material modifier, thus it is not retained and eluted first. For other proteins, the ones with lower pI have greater dissociation (increased negative charge). i.e., they are retained more strongly.
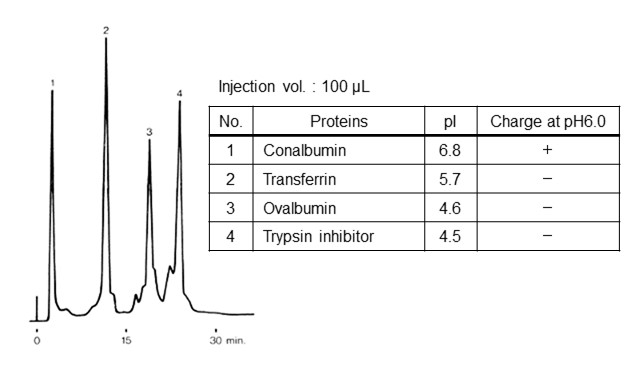
- Column
- :Shodex IEC QA-825 (8.0 mm I.D. x 75 mm)
- Eluent
- :(A); 20 mM Piperazine-HCl buffer (pH6.0)
(B); (A) + 0.5 M NaCl
Linear gradient; 0 min to 30 min, 100 % (A) to 50 % (B) - Flow rate
- :1.0 mL/min
- Detector
- :UV (280 nm)
- Column tamp.
- :Room temp.
Both polymer-based and silica-based packing materials are used for ion exchange mode columns. However, since alkaline solvents are sometimes used as an eluent, use of silica-based material is not as dominant as in reversed-phase mode columns, and use of polymer-based materials is relatively high in ion exchange mode. All Shodex ion exchange mode columns are polymer-based and we offer both anion exchange and cation exchange mode columns.
Find Shodex Anion Exchange Chromatography Columns HERE
Find Shodex Cation Exchange Chromatography Columns HERE
11-3. Ion chromatography and its separation mechanism
Ion chromatography is a type of ion exchange mode that mainly analyzes inorganic ions, halogen oxides, amines, and organic acids. Ion chromatography columns commonly have low ion exchange capacity and used with an electrical conductivity detector. There are two methods for the ion chromatography: suppressor and non-suppressor methods. Suppressor method is equipped with a device called a suppressor, which is placed between the column and the electrical conductivity detector, and the eluent eluted from the column is passed through the suppressor and then introduced into the electrical conductivity detector. With a non-suppressor method, eluent eluted from the column is directly introduced into the electrical conductivity detector. Details of conductivity detector will be explained in Chapter 5. 15-3 Conductivity detector. Similar to ion exchange mode, the elution sequence is from analytes with smaller to larger charge number or from larger to smaller hydrated ionic radius. Figure 21 shows an example analysis of inorganic anions, and Figure 22 shows an example analysis of inorganic cations.
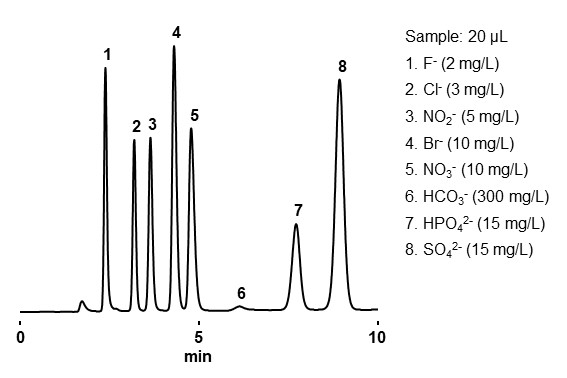
- Column
- :Shodex IC SI-90 4E (4.0 mm I.D. x 250 mm)
- Eluent
- : 1.8 mM Na2CO3 + 1.7 mM NaHCO3 aq.
- Flow rate
- :1.5 mL/min
- Detector
- :Suppressed conductivity
- Column tamp.
- :30 ℃
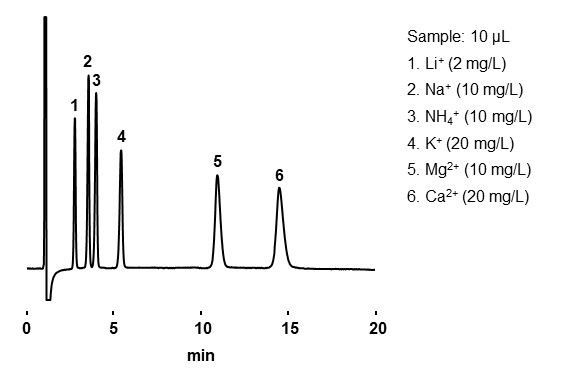
- Column
- :Shodex IC YS-50 (4.6 mm I.D. x 125 mm)
- Eluent
- :4 mM Methanesulfonic acid aq.
- Flow rate
- :1.0 mL/min
- Detector
- :Non-suppressed conductivity
- Column tamp.
- :40 ℃
Shodex offers columns for anion and cation analysis that are compatible with suppressor and non-suppressor methods.
Find Shodex Ion Chromatography Columns (Anion Analysis) HERE
Find Shodex Ion Chromatography Columns (Cation Analysis) HERE
11-4. Ion exclusion mode (ion exclusion chromatography) and its mechanism
Ion exchange mode uses attraction forces of ions between each other, whereas ion exclusion mode is a separation mode that uses repealing forces of ions between each other (electrostatic repulsion). A typical analysis where ion exclusion mode is effective is for the analysis of organic acids. The separation mechanism of ion exclusion mode will be explained using organic acid analysis as an example. In organic acid analysis, a packing material with a H-type, strongly acidic ion exchange group such as sulfo group is used. For an eluent, a strong acidic aqueous solution such as perchloric acid or sulfuric acid is used. The separation mechanism in ion exclusion mode is explained using a Donnan membrane, as shown in figure 23. The idea is that a virtual membrane called a Donnan membrane exists at the boundary between the functional group and the eluent, and the distribution occurs through this membrane. Strongly acidic ions such as chloride ions and sulfate ions completely dissociate into anions even in the acidic eluent. For this reason, electrostatic repulsion with functional group is large and it cannot pass through the Donnan membrane, and it flows through the column without being retained by the packing material. On the other hand, weak acids such as organic acids exist in an equilibrium state of dissociation and non-dissociation in the eluent. Undissociated organic acids pass through the Donnan membrane (distribute within the membrane), but when they dissociate into anions, they cannot pass through the Donnan membrane due to electrostatic repulsion. Therefore, organic acids with larger charges i.e., lower pKa (dissociation constant), are more difficult to pass through the Donnan membrane and elute faster. In addition, the organic acids that pass through the Donnan membrane also experience hydrophobic interactions with packing material, so less polar organic acids tend to elute slower. Figure 24 shows an example of organic acid analysis using ion exclusion mode. Shodex's organic acid analysis columns are packed with styrene divinylbenzene copolymer base material modified with sulfonic acid functional group (H-type).
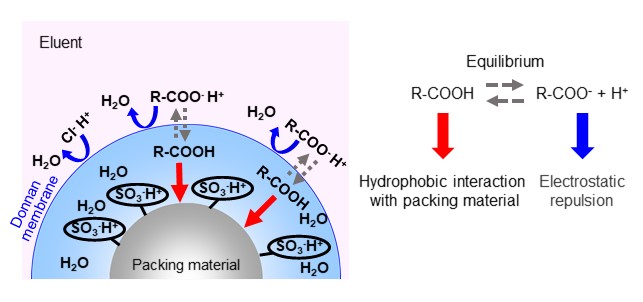
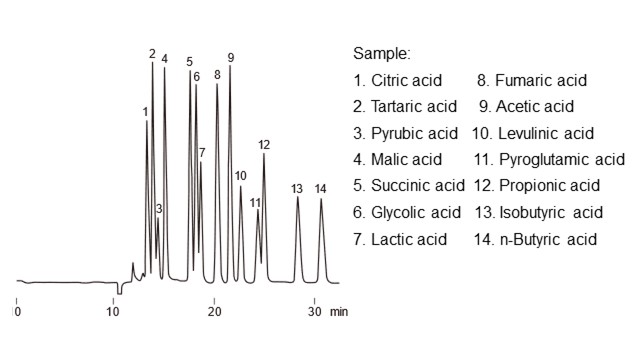
- Column
- :Shodex RSpak KC-811 (8.0 mm I.D. x 300 mm) x 2
- Eluent
- :6 mM HClO4 aq.
- Flow rate
- :1.0 mL/min
- Detector
- :VIS (430 nm) *Post column method
- Column tamp.
- :50 ℃
Find Shodex Ion Exclusion Chromatography Columns HERE
Table 4 summarizes ion exchange mode, ion chromatography, and ion exclusion mode.
Table 4 Comparisons of ion exchange mode, ion chromatography, and ion exclusion mode
Separation mode | Ion Exchange Mode | Ion Chromatography | Ion Exclusion Mode |
---|---|---|---|
Functional Group | Having high ion exchange capacity | Having low ion exchange capacity | Having strongly acidic ion exchange (H type) group |
Interaction | Eelectrostatic interaction | Electrostatic repulsion and hydrophobic interaction | |
Elution Sequence |
From smaller to larger electronic charge |
From smaller to larger pKa |
|
Target Analyte | Protein, peptide, nucleic acid etc. | Inorganic ions, halogen oxides, amines, organic acids, etc. | Mainly organic acids |